Introduction
The U.S. energy supply system is diverse and robust in its ability to provide a secure supply of energy with only occasional interruptions. However, projected impacts of climate change will increase energy use in the summer and pose additional risks to reliable energy supply. Extreme weather events and water shortages are already interrupting energy supply, and impacts are expected to increase in the future. Most vulnerabilities and risks to energy supply and use are unique to local situations; others are national in scope.
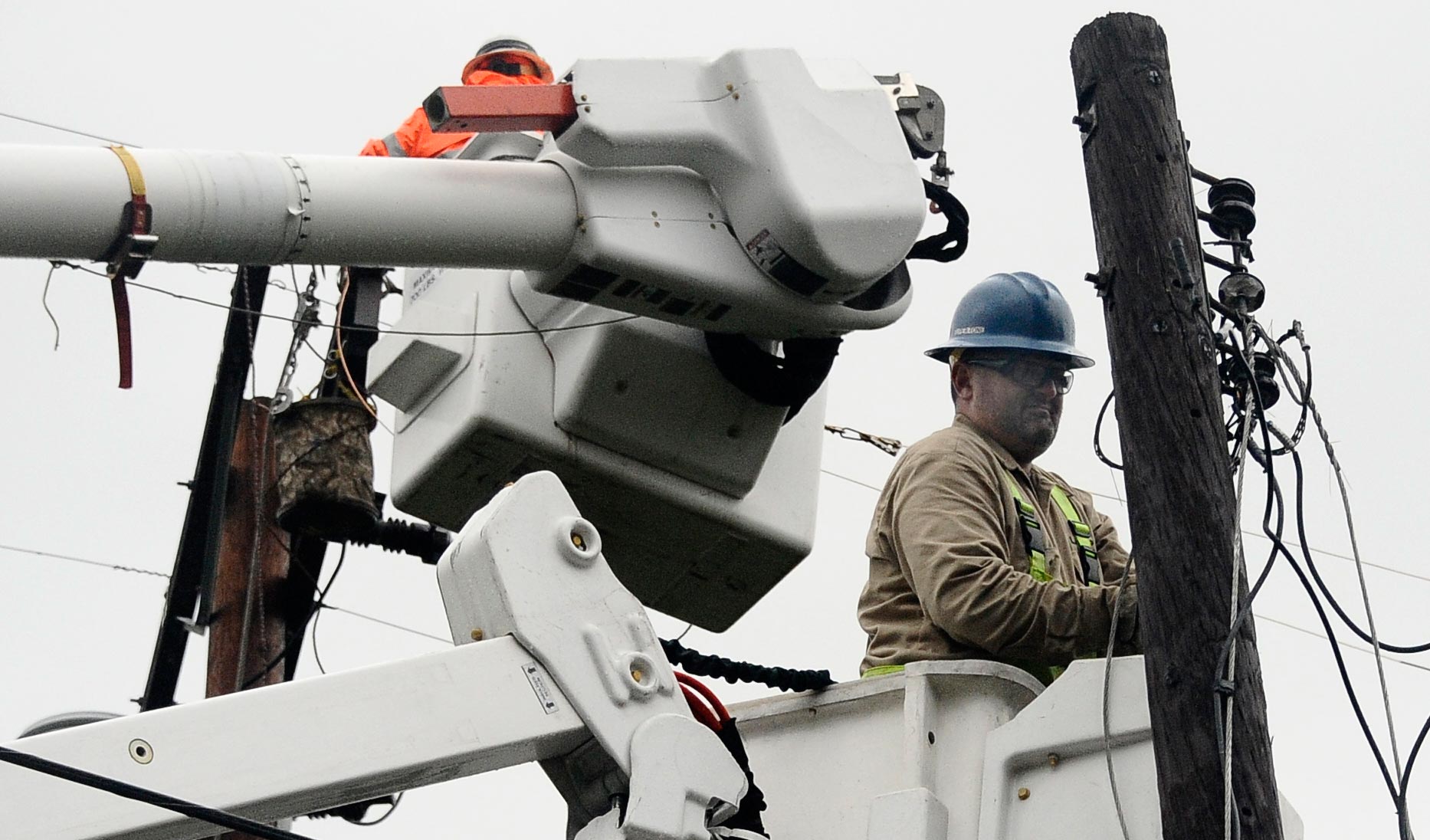
Energy infrastructure around the country has been compromised by extreme weather events
In addition to being vulnerable to the effects of climate change, electricity generation is a major source of the heat-trapping gases that contribute to climate change. Therefore, regulatory or policy efforts aimed at reducing emissions would also affect the energy supply system. See Ch. 10: Energy, Water, and Land, Key Message 2; and Ch. 27: Mitigation for more on this topic. This chapter focuses on impacts of climate change to the energy sector.
The impacts of climate change in other countries will also affect U.S. energy systems through global and regional cross-border markets and policies. Increased energy demand within global markets due to industrialization, population growth, and other factors will influence U.S. energy costs through competition for imported and exported energy products. The physical impacts of climate change on future energy systems in the 25- to 100-year timeframe will depend on how those energy systems evolve. That evolution will be driven by multiple factors, including technology innovations and carbon emission constraints.
Adaptation actions can allow energy infrastructure to adjust more readily to climate change. Many investments toward adaptation provide short-term benefits because they address current vulnerabilities as well as future risks, and thus entail “no regrets.” Such actions can include a focus on increased efficiency of energy use as well as improvements in the reliability of production and transmission of energy. The general concept of adaptation is presented in Chapter 28: Adaptation.