Introduction
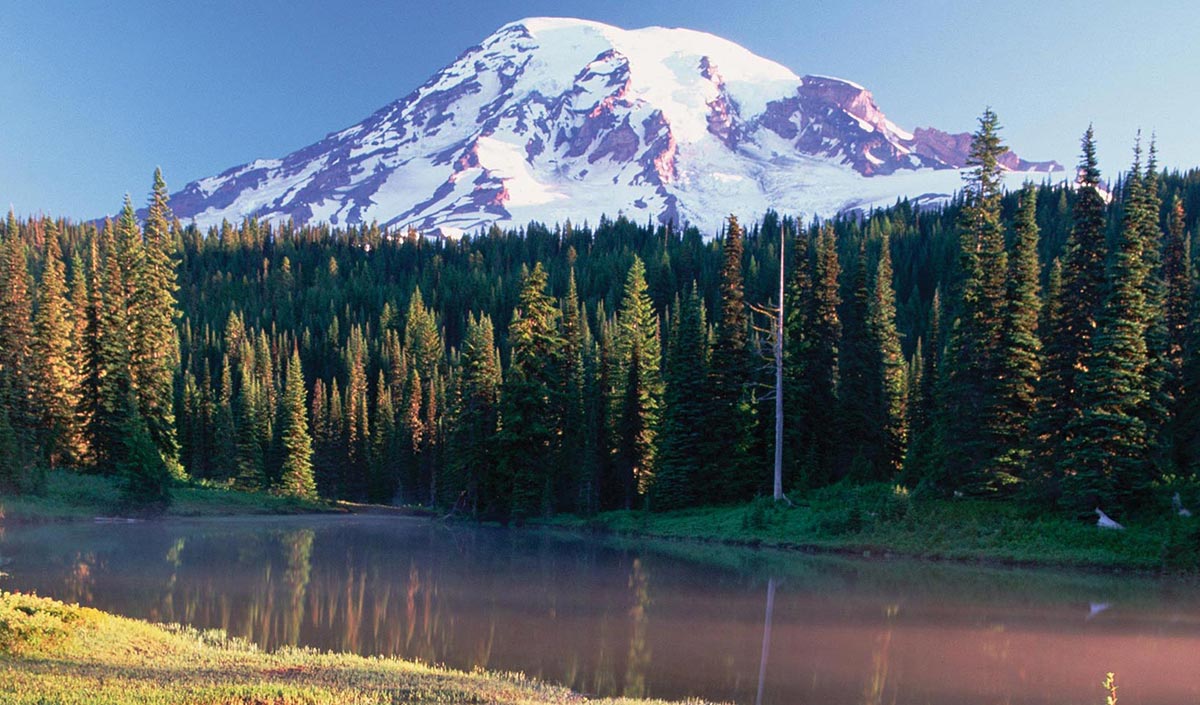
Changes in snowmelt patterns are affecting water supply.
Mt. Rainier, Washington.
Climate change impacts on biodiversity are already being observed in alteration of the timing of critical biological events such as spring bud burst, and substantial range shifts of many species. In the longer term, there is an increased risk of species extinction. These changes have social, cultural, and economic effects. Events such as droughts, floods, wildfires, and pest outbreaks associated with climate change (for example, bark beetles in the West) are already disrupting ecosystems. These changes limit the capacity of ecosystems, such as forests, barrier beaches, and wetlands, to continue to play important roles in reducing the impacts of extreme events on infrastructure, human communities, and other valued resources.
In addition to direct impacts on ecosystems, societal choices about land use and agricultural practices affect the cycling of carbon, nitrogen, phosphorus, sulfur, and other elements, which also influence climate. These choices can affect, positively or negatively, the rate and magnitude of climate change and the vulnerabilities of human and natural systems.